Ⅰ. Introduction
As the outermost organ in the human body, skin constantly faces the external environment and serves as a primary defense system. The protective functions of skin include UV-protection, anti-oxidant and anti-microbial functions1). The defects of epidermal barrier function is considered as a key event in allergic sensitization2). This study was carried out to investigate the effects of extracts of Anemarrhena asphodeloides (EAA) on skin barrier which has these important functions.
Intrinsic defects in skin barrier function and immune dysregulation resulting in Th2-predominant inflammation are closely associated with the pathogenesis of atopic skin inflammation3). With regards to immune abnormalities, atopic skin inflammation is currently considered as a biphasic T cell-mediated disease. Atopic skin inflammation is an imbalance in the Th1/Th2 immune response and IgE-driven inflammation. Th2 signal predominates in the acute phase, whereas Th2 to Th1 switch promotes disease in chronic phase. Moreover, disturbed skin barrier function such as that caused by mutations in the filaggrin gene (FLG) significantly increases the risk of atopic skin inflammation4,5).
Recently, herbal medicine have become the source of a large proportion of drugs and active compounds. Anemarrhena asphodeloides Bunge (Liliaceae) is a medicinal plant widely distributed in Korea, China, and Japan. The dried rhizomes of A. asphodeloides which contain steroidal saponins, xantones and polysaccharides are well-known traditional medicine used as an anti-depressant, anti-diabetics, anti-inflammatory, anti-platelet aggregation and anti-pyretic agent6,7).
Through the reports that A. asphodeloides water extract (AAWE) suppresses compound 48/80-induced mast cell activation by inhibition of cellular mechanisms in signaling pathways and would be beneficial for the treatment of mast cell-mediated anaphylactic response8), mangiferin isolated from A. asphodeloides exerts anti-photoaging activity in UVB-irradiated hairless mice9) and also attenuated hydrogen peroxide (H2O2) -induced MMP (matrix metalloproteinases) -1 activation related to oxidative stress causing skin aging, via inhibition of extracellular signal-regulated kinase (ERK) and Jun N-terminal kinase (JNK) pathway and AP complx-1 (AP-1)10), we can expect skin improvement effects of A. asphodeloides.
Although A. asphodeloides has been reported to show various biological activities, effects of ethanol (EtOH) extracts of A. asphodeloides (EAA) on skin barrier function remains unclear. In this study, we examined the effects of EAA on the skin barrier function in HaCaT keratinocytes.
Ⅱ. Material and Methods
Dulbecco’s modified Eagle’s medium (DMEM), fetal bovine serum (FBS), penicillin, and streptomycin were purchased from Life Technologies Inc. (Grand Island, NY, USA). 3-(4,5-dimethylthiazol-2-yl)-2,5-diphenyltetrazolium bromide (MTT), were purchased from Sigma Chemical Co. (St. Louis, MO, USA). inducible nitric oxide synthase (iNOS), filaggrin and β-actin monoclonal antibodies were purchased from Santa Cruz Biotechnology (Santa Cruz, CA, USA). Power SYBR® Green Master Mix was purchased from Applied Biosystems (Foster City, CA, USA). Glyceraldehyde 3-phosphate dehydrogenase (GAPDH), interleukin (IL)-4, IL-13, IL-6 and filaggrin oligonucleotide primers were purchased from Bioneer (Daejeon, Republic of Korea).
The roots of A. asphodeloides were purchased in Nanum herb (Yeongchen, Gyeongbuk, Korea). Dried roots of A. asphodeloides were extracted with 2ℓ 70% EtOH by maceration and the extracts were filtered. The filterate was evaporated in vacuo at 40℃, freeze-dried in vaccum. Finally, 65.72g of extract (at a concentration of 50㎍/㎖) was obtained from 200g of A. asphodeloides and stored at 20℃ for bioassays.
The HaCaT keratinocytes were provided by Prof. Seung-Heon Hong (Wonkwang University, Republic of Korea). The cells were cultured in DMEM supplemented with 10% FBS, penicillin (100U/㎖), and streptomycin (100㎍/㎖) in a 37°C and 5% CO2 incubator. Cells were treated with EAA (250 and 500㎍/㎖) for 1 hr. prior to stimulation with tumor necrosis factor-α (TNF-α)/interferon-γ (IFN-γ) (10g/㎖) for the indicated time.
HaCaT keratinocytes were plated at a density of 1×105 cells/well in 96 well plates. To determine the appropriate concentration of EAA which has no effect on cell viability, MTT assay was performed at 24 hrs. following treatment of EAA with various concentrations in HaCaT keratinocytes. Cell viabilities were measured at a 570㎚ by using Epoch® autometric microplate reader (Titertek Multiskan, Huntsville, AL).
Total RNA was isolated from cells by using the Easy-Blue® Reagent according to the manufacturer’s instructions Kits (Intron Biotechnology, Seongnam, South Korea). Total RNA was quantified by using an Epoch® autometric microplate reader (BioTek Instruments; Winooski, VT, USA). In addition, total RNA was converted to cDNA by using a high-capacity cDNA reverse transcription kit (Applied Biosystems; Foster City, CA, USA) and thermocycler (Gene Amp® PCR system 9700; Applied Biosystems) with the following program: initiation for 10 min. at 25℃, followed by incubation at 50℃ for 90 min. and at 85°C for 5 min. The size of the synthesized cDNAs was 200bp. qRT-PCR analysis was conducted by using a Step One Plus® Real-time PCR system (Applied Biosystems). SYBR® Green master mix and primers were used for PCR analysis of GAPDH, IL-4, IL-13, IL-6, and filaggrin. The PCR cycling parameters were as follows: 10 min. at 95℃; 40 cycles of 5 secs. at 95℃ and 45 secs. at 60℃; and a final melting curve of 15 secs. at 95℃, 1 min. at 60℃, and 15 secs. at 95℃. All primer sequences are shown in (Table 1). Gene expression was calculated according to the comparative threshold cycle (Ct) method. The results are expressed as the ratio of the optical density of the target locus to GAPDH.
The cells were re-suspended in protein extraction solution (PRO-PREP™, Intron Biotechnology, Seongnam, Republic of Korea) and incubated for 20 min. at 4°C. Cell debris was eliminated by micro-centrifugation, followed by immediate freezing of the supernatant. The protein supernatant concentration was measured by using the Bio-Rad protein assay reagent (Hercules, CA, USA) according to the manufacturer’s instructions. Each protein sample (30㎍) were electro-blotted transferred onto a polyvinylidene fluoride (PVDF) membrane followed by separation using 8–12% sodium dodecyl sulfate polyacrylamide gel electrophoresis. Membranes were incubated for 30 min. with blocking solution (2.5 or 5% skim milk) at room temperature, followed by incubation overnight with a 1:1,000 dilution of primary antibody at 4°C. Membranes were washed three times with Tris-buffered saline/ Tween 20 (TBS-T) and incubated with a 1:2,500 dilution of horseradish peroxidase-conjugated secondary antibody for 1 hr. 30 min. at room temperature. Membranes were again washed three times with TBS-T and then developed by enhanced chemiluminescence (Absignal™, Abclon, Seoul, Republic of Korea). The chemiluminescent blots were imaged on film. Densitometry analysis of the western blots was done by using ImageJ software (National Institutes of Health, Bethesda, MD). The intensity of the individual bands on western blot analysis was measured by gel analyzer tools of ImageJ and normalized either to β-actin to its total protein. The graphs represent normalized values as fold change over control condition.
HaCaT keratinocytes cells were seeded into 24-well plates (1 × 105 cells/well). After 24 hrs. of incubation, the monolayers were scratched with sterile pipette tips and treated with or without EAA (250 or 500㎍/㎖). After 72 hrs., the cells were fixed in 4% formalin for 20 min. The wound closure distance was evaluated using an Eclipse TE2000U inverted microscope with twin CCD cameras (magnification, ×200; Nikon, Tokyo, Japan; n = 3).
All the values reported have been expressed as the mean ± standard deviation (SD). Data were analyzed using one-way analysis of variance (ANOVA) with Dunnett’s test, and p-values < 0.05 were considered statistically significant. All statistical analyses were performed using GraphPad Prism (version 5.00 for Windows, San Diego, CA, USA).
Ⅲ. Results
To measure the cytotoxic effects of EAA on HaCaT keratinocytes, cell viability was investigated by using MTT assay. Treatment with different concentration of EAA ranging from 7.8 to 500㎍/㎖ for 24 hrs. did not have any cytotoxic effects in HaCaT keratinocytes(Fig. 1). Thus, we used EAA at a concentration of 250 and 500㎍/㎖ for subsequent experiments.
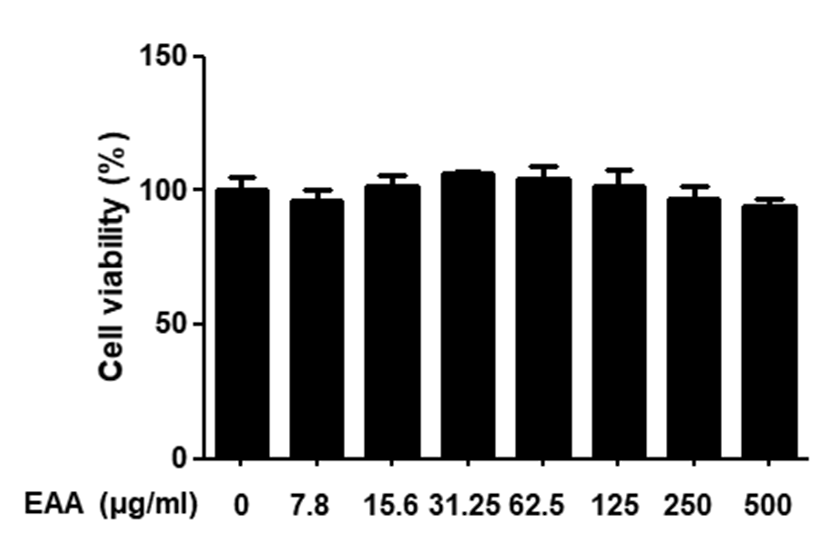
Epidermis, the outermost layer of the skin, consists of stratified squamous epithelium which differentiates from proliferating basal keratinocytes11). During wound re-epithelialisation stage, skin lesions close the wound and remodel the cytoskeleton by enhancing the proliferation and migration of keratinocytes12). To evaluate whether EAA can influence keratinocytes migration, we assessed the effects of EAA on HaCaT keratinocytes motility using wound healing assay. The migration of HaCaT keratinocytes was evaluated by wound closure distance. Treatment of HaCaT keratinocytes with EAA for 24 hrs. increased the migration of keratinocytes(Fig. 2).
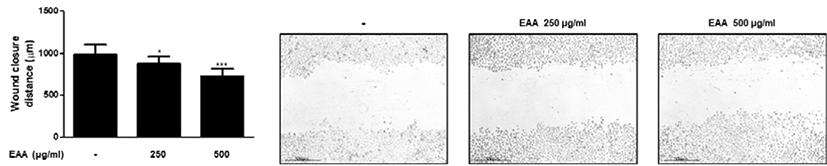
iNOS mediates several inflammatory responses by the overproduction of Nitric oxide (NO) and is induced in response to various pro-inflammatory stimuli such as TNF-α, IFN-γ, IL-6, and lipopolysaccharide (LPS)13). In addition, NO is thought to be a key disruptive factor in the wound healing process12). Because iNOS is highly involved in the inflammatory responses, we examined the effects of EAA on the level of iNOS protein expressions by western blot analysis. The expression levels of iNOS proteins were significantly up-regulated in response to TNF-α/IFN-γ co-stimulation, while pre-treatment with EAA inhibited the expression levels of iNOS proteins in a dose-dependent manner(Fig. 3).
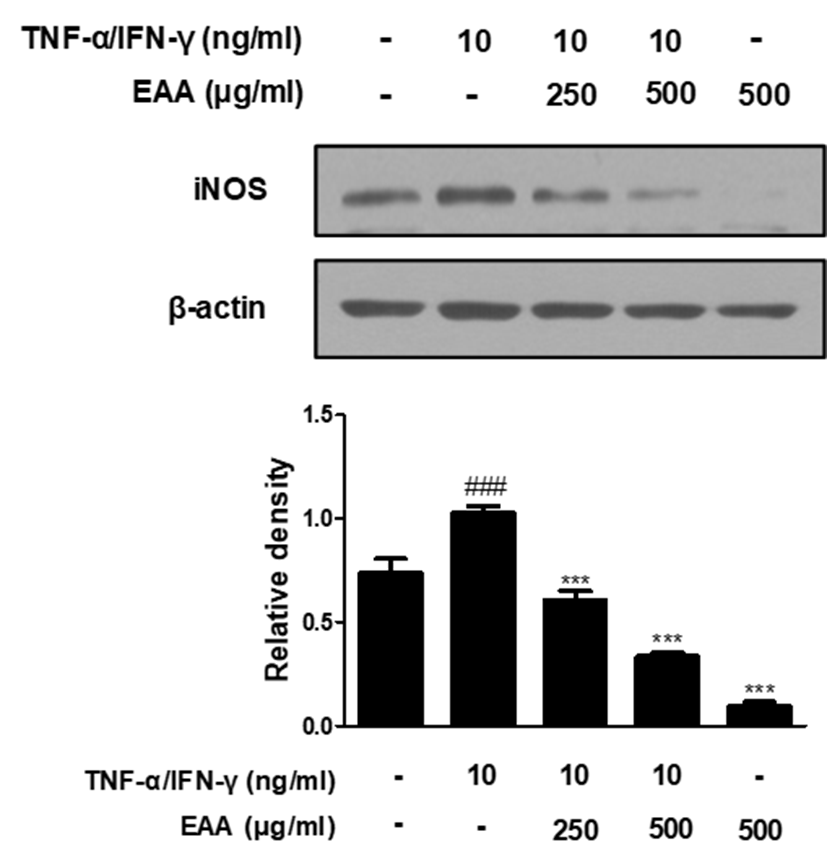
Atopic skin inflammation is a kind of cutaneous inflammation reaction characterized by Th2 cell-predominant phenotype in the initial stage with the additional acquisition of Th1 cell phenotype during the chronic phase3). IL-4 has previously shown to be elevated in the skin from patients with atopic skin inflammation14). IL-4 plays an important role in switching to IgE isotype by differentiating B cells and inhibits the proliferation and differentiation of Th1 cells. Also, IL-4 promotes the differentiation of naive T cells into allergic type Th2 cells15). In TNF-α/IFN-γ-stimulated HaCaT keratinocytes, to elucidate the effects of EAA treatment on cytokine expression, alterations in the mRNA expression levels of various cytokines were measured using qRT-PCR. TNF-α/IFN-γ significantly increased the mRNA expression of IL-4 in HaCaT keratinocytes. However, pre-treatment with EAA significantly suppressed the mRNA expression of IL-4(Fig. 4).
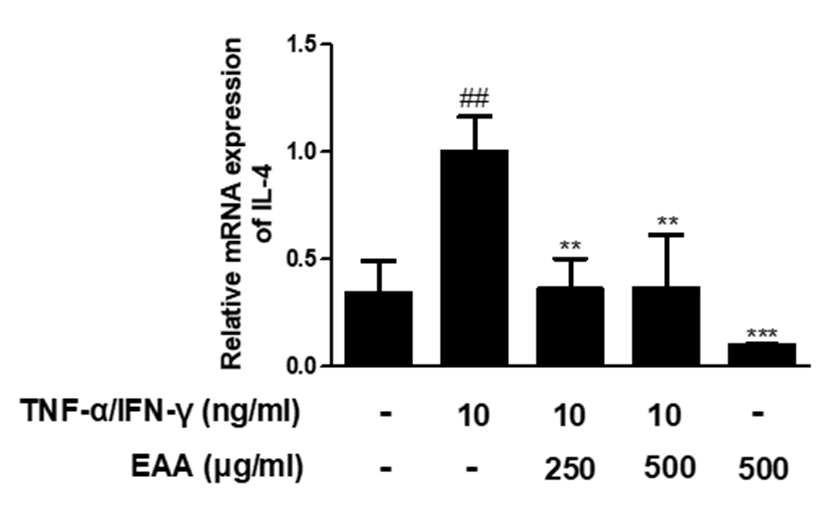
It is well known that the levels of Th2 type cytokines are elevated in the skin of atopic skin inflammation patients and IL-13 levels are further increased in patients with elevated IgE levels16). IL-13 as well as IL-4, induce B cell proliferation and immunoglobulin class switching for IgE production17). EAA pre-treatment in TNF-α/IFN-γ-stimulated HaCaT keratinocytes reduced IL-13 mRNA expression, compared to TNF-α/IFN-γ-stimulation(Fig. 5).
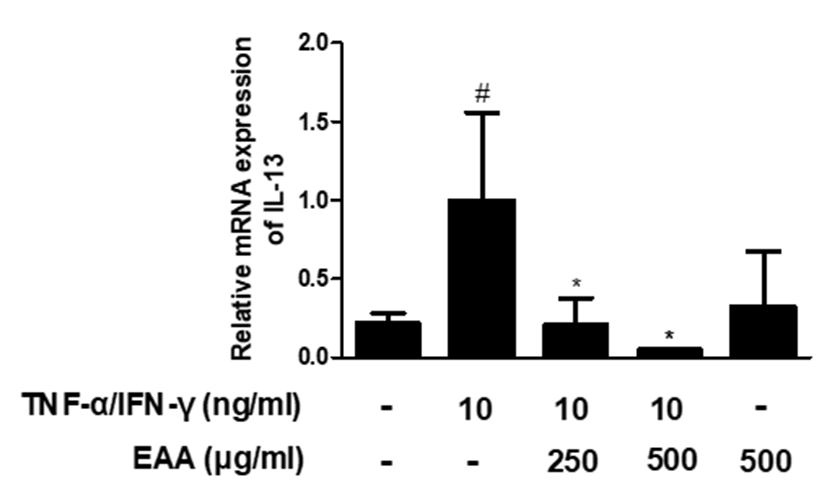
IL-6 is one of pro-inflammatory cytokines that regulates Th2 differentiation and inhibition, as well as CD4+ T cell differentiation. Also, IL-6 is known as negative regulator of epidermal differentiation18,19). TNF-α/IFN-γ significantly increased the mRNA expression of IL-6 in HaCaT keratinocytes. However, pre-treatment with EAA significantly suppressed the mRNA expression of IL-6 in dose-dependent manner(Fig. 6). These results suggest that EAA has protective effects on skin keratinocytes by inhibiting the transcriptional level of inflammatory cytokines related to skin barrier dysfunction.
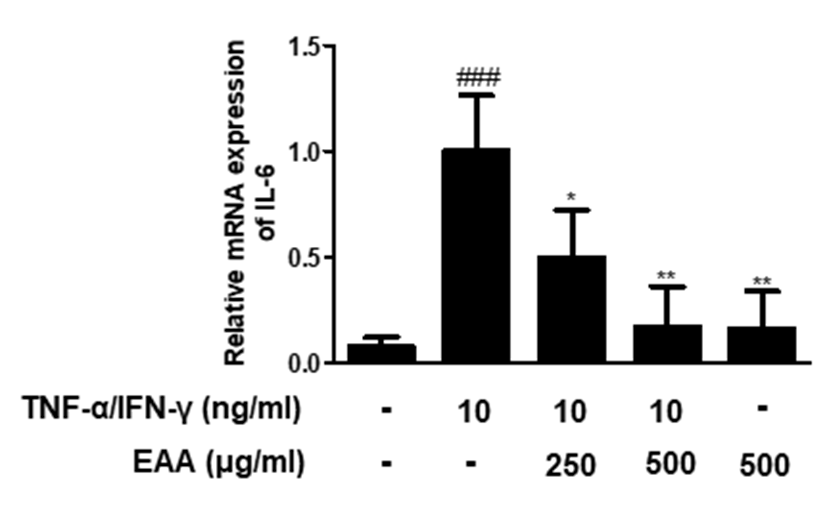
Filaggrin is a key protein that plays an important role in formation of the cornified cell envelope which is critical for an effective skin barrier20). We investigated the effects of EAA on the protein and mRNA expression levels of filaggrin by using western blot analysis and qRT-PCR. TNF-α/IFN-γ co-stimulation decreased the protein and mRNA expression levels of filaggrin, but pre-treatment with EAA significantly increased the filaggrin protein level, although the mRNA level was increased slightly(Fig. 7). These results suggest that EAA has the effects of restoring filaggrin in TNF-α/IFN-γ-stimulated HaCaT keratinocytes.
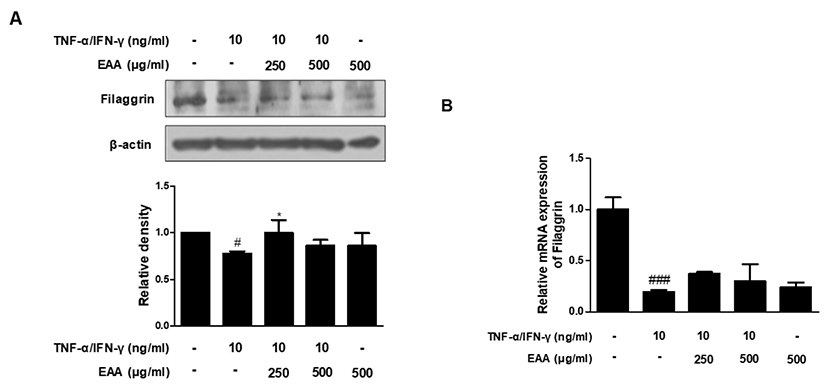
Ⅳ. Discussion
The skin plays a major role in protection from the external environment. Stratum corneum prevents transepidermal water loss and protects the skin from external environment21). Among these important functions, defensive functions can be further classified as physical, thermal, immune, ultraviolet, oxidant, racial, anti-microbial and the permeability barrier1). In 1975, Michaels et al. suggested a schematic model to explain the permeability of the stratum corneum, called ‘brick and mortar’ where the corneocytes are the bricks and the lipids are the mortar22). Today this is viewed as the most appropriate model for the understanding of cellular arrangement and the winding ways of skin permeability23).
The skin consists of epidermis, dermis and subcutaneous fat. Among the cells known to be involved in atopic dermatitis, keratinocytes constitute more than 95% of all the epidermis. keratinocytes form keratin and produce cytokines, chemokines by various stimuli and also express various receptors on the cell surface24). This barrier between the body and the environment is constantly maintained by reproduction of inner living epidermal keratinocytes which undergo a process of terminal differentiation and then migrate to the surface as interlocking layers of dead stratum corneum cells. These cells provide the bulwark of mechanical and chemical protection and together with their intercellular lipid surroundings, confer water-impermeability25). Defects of the skin barrier function lead to the increased transepidermal water loss and increased penetration of harmful agent. Consequently, it allows inhaled substances such as microbes, allergens/antigens and irritants to pass more easily into the dermis to interact with immune and inflammatory cells and possibly contributes to the Th2 immune response as a common feature in many inflammatory diseases including inflammatory bowel disease, celiac disease, sinusitis, food allergy, asthma and atopic dermatitis21,26,27).
The very early event initiating atopic skin inflammation is remain unclear. However, one of the central causes of the atopic skin inflammation is the dysregulated Th1 and Th2 response that induces the characteristic Th2-dominant skin allergic inflammation and the production of primary pro-inflammatory cytokines after skin barrier disruption is a well-accepted script24,28). In atopic skin inflammation patients, genetic conditions, external stimuli or scratching episodes disrupt skin barrier, which facilitates allergen penetration and activation of keratinocytes to produce chemokines that trigger dendritic cells to induce Th2-cell mediated response. In the acute phase of disease, infiltrated CD4+ T cells in skin lesions predominantly secrete IL-4, IL-5, and IL-1329). Increased Th2 cytokine expression contributes to reduction in anti-microbial peptides and reduced filaggrin expression. For instance, IL-4, a Th2 cytokine, suppresses the enhancement of ceramide synthesis and cutaneous permeability barrier function induced by TNF-α and IFN-γ in human epidermal sheets. In addition, IL-4 blocks the recovery of barrier function and enhancement of ceramide synthesis after barrier disruption by acetone treatment in living skin equivalent30). In this study, EAA suppressed the mRNA levels of IL-4, IL-13, and IL-6 in TNF-α/IFN-γ-stimulated HaCaT keratinocytes. These results show that EAA has inhibitory effects on skin barrier disruption by suppressing pro-inflammatory cytokines.
Cutaneous wound healing is a dynamic and complex process involving intricate interactions among a variety of inflammatory cells, extracellular matrix molecules, parenchymal cells and soluble molecules. The wound healing process consists of three steps: inflammatory, proliferative and remodelling31). The proliferative phase of wound healing is associated with the re-epithelialisation process including collagen production and extracellular matrix molecules remodelling. Skin lesions close the wound and remodel the cytoskeleton by enhancing the proliferation and migration of keratinocytes for wound re-epithelialisation stage. During the early stage of wound healing, collagen production is promoted to increase scar formation, and then it is gradually decreased to normal levels in the final stage of wound healing12). NOS catalyzes the synthesis of a free radical NO and l-citrulline from l-arginine. Especially, NO has many physiological functions such as vasorelaxation, neurotransmission, inflammation and wound healing32). NO is a highly reactive radical that is generated by the activation of iNOS and contributes to various biological processes including inflammation. On the other hand, NO is thought to be a key disruptive factor in the wound healing process, although it was reported that small amounts of NO may enhance wound healing during the early stage12). Likewise, excessive generation of Prostaglandin E2 (PGE2) by cyclo-oxygenase-2 (COX-2) is a crucial physiological factor contributing inflammation development33). In this study, it is found that treatment with EAA increased the migration of HaCaT keratinocytes and inhibited expression levels of iNOS proteins. It is possible to assume that EAA facilitates the wound healing in skin barrier through inhibition of excessive expression of NO.
Filaggrin is a filament-associated protein derived via dephosphorylation from profilaggrin which is the major component of keratohyalin granules within epidermal granular cells. The primary function of filaggrin is modulation of epidermal homeostasis via interaction with keratin filaments3). Filaggrin is initially expressed as an inactive precursor protein, profilaggrin which is released from the keratohyalin F granules that are visible in the granular cell layer of the epidermis 34). The primary role of filaggrin is to facilitate the bundling of the keratin cytoskeleton to form macrofibrils which functions as a scaffold for subsequent reinforcement stages of stratum corneum assembly. The disordered profilaggrin processing results in the complete absence of filaggrin monomers and abnormal lipid formation resulting in a lethally impaired skin barrier35). The expression of barrier protein genes, such as filaggrin and loricrin is up and downregulated by various external and internal stimuli, including Th2 (IL-4 and IL-13) cytokines. The loss of mutation function of filaggrin in atopic skin inflammation, the upregulation of Th2 cytokines explain the disrupted expression of filaggrin and loricrin in the lesional skin of atopic skin inflammation patients, leading to epidermal barrier dysfunction36). In this study, it is observed that filaggrin protein level was restored by EAA pre-treatment in TNF-α/IFN-γ-stimulated HaCaT keratinocytes.
Ⅴ. Conclusions
In this study, we determined the mechanisms of the protective effects of EAA on skin barrier function in TNF-α/IFN-γ stimulated HaCaT keratinocytes.
1. The inhibitory effects of EAA is observed in the expression of Th2 type cytokine, such as IL-4 and IL-13 and pro-inflammatory cytokine such as IL-6 at mRNA expression levels.
2. EAA increased the migration of HaCaT keratinocytes.
3. EAA inhibited expression levels of iNOS proteins.
4. EAA increased level of filaggrin at protein and mRNA expression.
These results show that EAA has inhibitory effects on skin barrier disruption due to inflammation by suppressing Th2 type cytokine, pro-inflammatory cytokine and by filaggrin upregulation consequently. In conclusion, these data suggest that EAA is a source that can be considered as a potent candidate for the improvement of skin barrier function for external use.